In this article, we will try to answer the following questions:
1. What makes dental implants biocompatible?
2. What can improve biocompatibility?
3. What are the potential downsides?
Let us start with a bit of history:
Our bodies do not reject titanium. The discovery is attributed to Per‐Ingvar Branemark, a Swedish physician who implanted a titanium screw into a bunny bone and reported no rejection by the surrounding tissue (1). To use Hollywood jargon in regards to animals in movies: “The bunny was seriously hurt in the process of ‘filming’ the 1969 paper”.
How did he come up with the idea to use a titanium screw, one may rightfully ask? This is because there was some previous knowledge about titanium’s properties published before World War II. The researchers implanted various metals into lab animals’ bones and found that titanium was “special” in a good way (2). The discovery is not attributed to Bothe, Beaton, and Davenport, who published this, but rather to the person who did more or less the same thing 29 years later.
Branemark didn’t get recognition right after his discovery. He tried to secure funding for his research but couldn’t win grants from his own people. The scientific community couldn’t accept that a metal could be biocompatible. Luckily, NIH gave him a grant to continue his research, and finally, in 1982, he demonstrated to the American Dentist Association that titanium teeth implants work (3). His research didn’t result in a Nobel Prize, regardless of the fact that he was of the right gender and the right ancestry for the time period. His discovery apparently didn’t seem original enough to the Nobel Committee. Since he was at the epicenter of action (Sweden), one can’t help but speculate that the decision-makers knew him and didn’t like something about him, which is always a factor when impartial decisions are made. Well, he did file for many patents in the early days of implant surgery. Not bad, really. If something was hurt, it was probably just the ego.
What makes titanium biocompatible?
Titanium is not inert at all. It reacts with the surrounding tissues, but it seems that the body doesn’t mind it. The important feature of this metal is its ability to coat its surface with a thin layer of oxide, thus preventing corrosion. When titanium is exposed to body fluids, the oxide on the surface becomes hydrated, so the surface is covered with Ti-OH– molecules that are negatively charged, attracting Ca2+ ions that lead to the formation of apatite layer as there are phosphate ions around as well. Hydroxyapatite is a natural component of bones; hence, it is biocompatible. This is a somewhat simplified explanation, but the basic mechanism is correct. We believe in not overwhelming the readers with details right at the beginning because once the underlying mechanism is understood, it is easy to fill in the details later.
We can now go into details:
The process is not as simple as if a smooth, shiny metal were drilled into the bone. In fact, the surface of the titanium screw is heavily modified. Take a look at the image. The screw part of the implant is titanium, but it looks white and rough because of the oxide layer. To achieve this effect, implant manufacturers use different approaches. There are two most common metal treatments: SLA and PSC.
SLA stands for “Sand blast Larger grit and Acid etching” (4). The treatment starts with large abrasive particles (5-20 microns), then acid treatment, after which another sandblasting with a particle range of 3-5 microns is applied, followed by another treatment with sulfuric acid. The specifics of the protocol were probably developed based on empirical evidence, as electron microscopy showed that osteoblasts prefer to colonize a uniformly etched surface as opposed to an irregular one. This uniformity is achieved with an acid treatment. Sandblasting alone produces irregular surface and it’s not enough.
Another very common surface treatment is Plasma Spray Coating. Materials such as hydrohyapatite are heated to high temperatures (9000 °C) and deposited on the surface of the implant. Titanium can also be deposited on the surface as well. Since the goal is to achieve adequate roughness and uniformity, the initially strange-sounding idea of depositing titanium on titanium eventually makes sense. Plasma coating is used more frequently than the SLA method.
The previous two methods started with a smooth metal surface. There is an alternative approach. One can produce a porous titanium implant because it can improve osseointegration (colonization by osteoblasts). The downside is a messy surgery if it ever needs to be removed.
The efficiency of the surface treatments is evaluated with Simulated Body Fluids solution. As the name suggests, this solution mimics the environment of the body. It was shown that plasma-coated hydrohyapatite is less durable and makes less contact with the tissue than titanium coating (4,5). This is strange because it is the natural bone material, so could it be that the result was observed due to the technique itself, not the intrinsic quality of the material used? Our bones are not titanium-strong. Hydrohyapatite should be strong enough and more readily accepted than the foreign metal. Strange results…
After the surface of an implant is treated with SLA or PSC, the implant is soaked in sodium hydroxide. This step enhances the production of amorphous sodium titanate in the form of a hydrogel. Once heated, it dries, becoming more dense, which is desirable. The surface will be more hydrophilic once it is back in the solution. Surface “wetting” is essential for the integration of an implant into the surrounding tissue. Material that is hydrophobic or inert will not get coated with body fluids and will not attract osteoblasts for colonization.
So far, we have discussed only the chemistry of the process, not biology or timing. Within 24 hours of the surgery, the implant site is populated only by red blood cells and macrophages (6). After 96 hours, the site is visited by mesechymal stem cells. New blood vessels are being formed. After four weeks, osteoblasts colonize the area and finally bridge the implant with the surrounding tissue after approximately six to eight weeks.
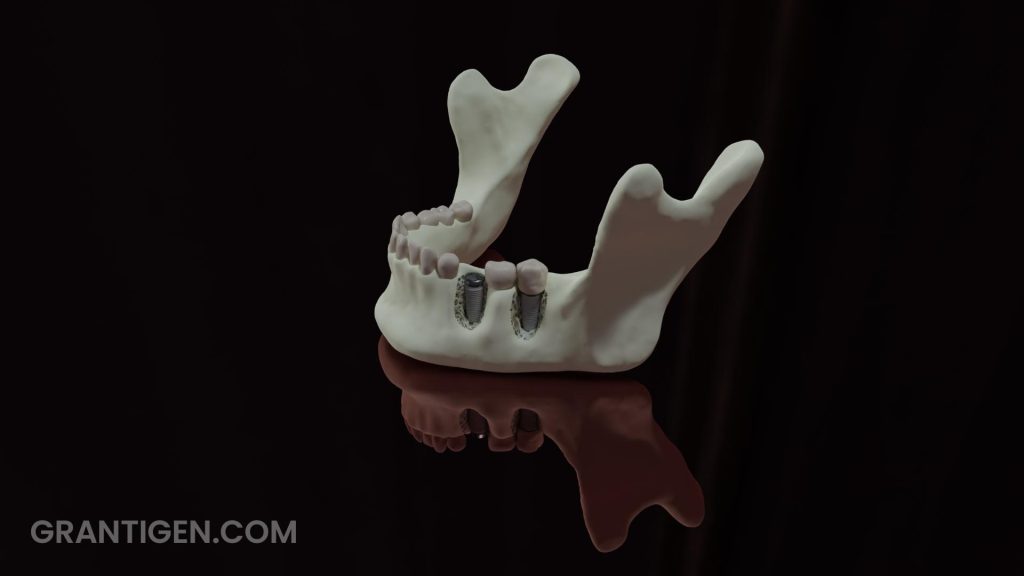
Zirconia as a titanium alternative:
Zirconium belongs to the same group as titanium in the periodic table. Their properties should be similar. Still, metal zirconium is not used for manufacturing implants but rather zirconium dioxide or zirconia. Being a white ceramic material, it has the advantage of appearance, but titanium shows better mechanical properties (7). In terms of biocompatibility, they are comparable, provided that proper surface treatment has been applied. With no prior surface treatment, titanium is superior, but that might be a moot point.
What can be done to improve the biocompatibility of implants?
There seem to be no additional candidates that can replace titanium or zirconia for now. For that reason, improvements to surface treatment methods will most likely be a major thing in the near future. One thing that is important but has not been discussed so far is bacterial infection. If the implant does not adhere well to the surrounding tissues, bacteria will, and once they form a biofilm, they can cause severe infections, leading to the loss of the implant. Preventing bacterial infections is one direction that may improve the longevity of implants. An elegant method has been devised. It consists of doping titanium with copper (8). Small amounts of copper are not toxic to us, but localized release at the implant site prevents bacterial growth. Neat!
What are the downsides?
This world would not be as it is if there were no data in direct opposition to what we have said so far. New research shows that both titanium and titanium dioxide might be toxic. The first thing that comes to mind is silica. If you need pure silica for the lab, it will come in a container with a label saying “carcinogen”. People who do metal casting use fine silica, and they are in real danger of developing silicosis and lung cancer (9). Still, we don’t wear a mask once we go to the beach. This statement has been challenged lately by some Darwin Award candidates.
We have had decades worth of data that shows one thing, and now we have some new, potentially deeper insights into why the previous observations were not perfect. The potential harmful effects of TiO2 are more related to the large amounts of nanoparticles that we use as a food additive and sunscreen (10). The amounts of titanium dioxide on the surface of an implant are tiny in comparison to what is used in food or cosmetics, but the negative effects do come from the metal itself.
One downside of titanium implants is hypersensitivity in some patients (11). A patch test used to determine allergy sounds logical, but it doesn’t correlate with the clinical outcome (12).
In addition to allergies, titanium can cause the nails to turn yellow – the yellow nail syndrome (13). There have been reports of the accumulation of titanium in organs and chronic sinusitis. The main toxicity- and allergy-producing components are the metals used to make the titanium alloy, such as aluminum and vanadium. These metals are common in modern titanium implants. For an in-depth review of titanium toxicity, we recommend the following reference (12). Someone who has no knowledge of metallurgy would now ask, “But why don’t they use pure titanium?”. No, it is not practical. Working with pure metals (other than gold) is a pain unlike many others. Pure metals crystallize and have a very sharp melting point that is usually higher than when alloyed. Machining is more difficult because pure metals tend to be brittle, so alloys are always better. In this particular case, the metals added to titanium should be tissue-friendly. Most are not.
Manufacturers claim that the implant can last 25 years or even longer and that the failure is due to bone loss because implants are usually used in advanced-age patients. It would be interesting to see a study with individuals who had dental implants much earlier in life.
References:
1. Branemark PI, Adell R, Breine U, Hansson BO, Lindstrom J, Ohlsson A. Intra-osseous anchorage of dental prostheses. I. Experimental studies. Scand J Plast Reconstr Surg. 1969;3(2):81-100. doi: 10.3109/02844316909036699. PMID: 4924041.
2. Jokstad A. Why did Professor Per-Ingvar Brånemark never receive the Nobel Prize in Medicine? Clin Exp Dent Res. 2017 Jul 3;3(3):79-80. doi: 10.1002/cre2.72. PMID: 29744182; PMCID: PMC5719826.
3. Kim TI. A tribute to Dr. Per-Ingvar Brånemark. J Periodontal Implant Sci. 2014 Dec;44(6):265. doi: 10.5051/jpis.2014.44.6.265. Epub 2014 Dec 31. PMID: 25568805; PMCID: PMC4284373.
4. Jemat A, Ghazali MJ, Razali M, Otsuka Y. Surface Modifications and Their Effects on Titanium Dental Implants. Biomed Res Int. 2015;2015:791725. doi: 10.1155/2015/791725. Epub 2015 Sep 7. PMID: 26436097; PMCID: PMC4575991.
5. Yang GL, He FM, Yang XF, Wang XX, Zhao SF. Bone responses to titanium implants surface-roughened by sandblasted and double etched treatments in a rabbit model. Oral Surg Oral Med Oral Pathol Oral Radiol Endod. 2008 Oct;106(4):516-24. doi: 10.1016/j.tripleo.2008.03.017. Epub 2008 Jul 7. PMID: 18602288.
6. Silva RCS, Agrelli A, Andrade AN, Mendes-Marques CL, Arruda IRS, Santos LRL, Vasconcelos NF, Machado G. Titanium Dental Implants: An Overview of Applied Nanobiotechnology to Improve Biocompatibility and Prevent Infections. Materials (Basel). 2022 Apr 27;15(9):3150. doi: 10.3390/ma15093150. PMID: 35591484; PMCID: PMC9104688.
7. Hanawa T. Zirconia versus titanium in dentistry: A review. Dent Mater J. 2020 Jan 31;39(1):24-36. doi: 10.4012/dmj.2019-172. Epub 2019 Oct 30. PMID: 31666488.
8. Wu Y, Zhou H, Zeng Y, Xie H, Ma D, Wang Z, Liang H. Recent Advances in Copper-Doped Titanium Implants. Materials (Basel). 2022 Mar 22;15(7):2342. doi: 10.3390/ma15072342. PMID: 35407675; PMCID: PMC8999642.
9. Sato T, Shimosato T, Klinman DM. Silicosis and lung cancer: current perspectives. Lung Cancer (Auckl). 2018 Oct 26;9:91-101. doi: 10.2147/LCTT.S156376. PMID: 30498384; PMCID: PMC6207090.
10. Racovita AD. Titanium Dioxide: Structure, Impact, and Toxicity. Int J Environ Res Public Health. 2022 May 6;19(9):5681. doi: 10.3390/ijerph19095681. PMID: 35565075; PMCID: PMC9104107.
11.Poli PP, de Miranda FV, Polo TOB, Santiago Júnior JF, Lima Neto TJ, Rios BR, Assunção WG, Ervolino E, Maiorana C, Faverani LP. Titanium Allergy Caused by Dental Implants: A Systematic Literature Review and Case Report. Materials (Basel). 2021 Sep 12;14(18):5239. doi: 10.3390/ma14185239. PMID: 34576463; PMCID: PMC8465040.
12. Kim KT, Eo MY, Nguyen TTH, Kim SM. General review of titanium toxicity. Int J Implant Dent. 2019 Mar 11;5(1):10. doi: 10.1186/s40729-019-0162-x. PMID: 30854575; PMCID: PMC6409289.
13. Decker A, Daly D, Scher RK. Role of Titanium in the Development of Yellow Nail Syndrome. Skin Appendage Disord. 2015 Mar;1(1):28-30. doi: 10.1159/000375171. Epub 2015 Feb 11. PMID: 27172293; PMCID: PMC4857837.